No one is glad when their car breaks down. It is a major inconvenience and repairs can be expensive. But what if your car could sense wear and tear, and strengthen itself in those areas to prevent further damage? The car would last longer, require fewer repairs, and be less likely to fail when it counts. This might sound like science fiction, but self-strengthening technology could become a reality in the not-too-distant future.
Sophia Huang, a PhD candidate in the Esser-Kahn Group at the University of Chicago, is currently working to create a material that strengthens itself in response to mechanical force and does so by exploiting the mechanical force itself.
But even small amounts of mechanical force (any force used to push or pull, apply pressure, shake or deform) can cause damage when applied over and over. So how could a source of damage be harnessed to make an object stronger?
Turning force into strength
Tiny particles called piezoelectric nanoparticles, or piezo-NPs, could be the key to bringing self-strengthening materials into reality.
When a mechanical force is inflicted on a material in which piezo-NPs are embedded, the piezo-NPs send electrical signals. Those signals stimulate surrounding molecules, causing them to form chemical bonds with each other. These molecular connections make the material stronger and thus more resistant to damage in the future.
But there is a reason piezo-NPs are not already being used to prevent car troubles. So far, Huang and her research group have only been able to demonstrate self-strengthening in a type of gel. This gel looks and feels a little like Jell-O and, as Huang describes, has some limitations: “It’s not really mechanically robust, so you can’t really make it into a ton of stuff. You can’t make it into a phone, you can’t make it into a vehicle.”
So why hasn’t she made a more rigid material? Because it’s easier to make a self-strengthening gel than a self-strengthening solid.
Gels are mostly liquid, and molecules in liquids can move around much more easily than they can in solids. When molecules are free to move around, the signals from the piezo-NPs can travel far enough to trigger those strengthening bonds. But in most rigid materials, the signals do not travel as far and few, if any, self-strengthening chemical bonds are formed.
Huang is now experimenting with different ingredients for the gel, to find ones that allow the signals from the piezo-NPs to travel relatively long distances. She can then study the chemical properties of those ingredients and identify rigid materials with similar properties that would let the piezo-NPs’ self-strengthening signals propagate further.
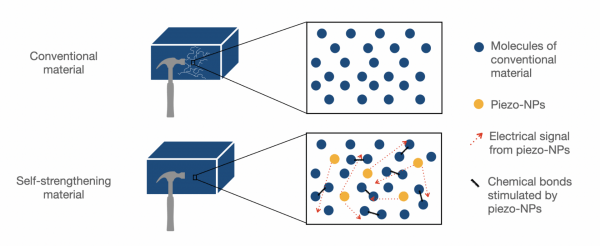
Conventional materials weaken under force, while the molecules in self-strengthening materials send signals to form chemical bonds, making the materials stronger.
Creating a new material, one experiment at a time
Huang has big ideas for how self-strengthening technology could be applied if it could be used in more rigid materials. She names a wide variety of potential applications, including car engines, undersea pipelines, space shuttles, and even biomedical implants like artificial joints and pacemakers.
“I think applications where self-remodeling materials will really shine are things that are difficult, costly, or impossible to maintain or repair, but that experience consistent mechanical vibration from normal use,” Huang said. “In those cases, self-remodeling materials will help prevent catastrophic incidents.”
The self-strengthening gel itself might not be strong enough to build all that, but understanding its underlying physics and chemistry will help guide the production of more robust materials.
Recently, Huang has focused on testing the properties of the liquid part of the gel. For each experiment, she uses a different chemical in liquid form to soak a piezo-NP loaded gel, and then tests that gel for how well electrical signals from the piezo-NPs can travel through it.
To activate the piezo-NPs in an experiment, the gel must receive a mechanical force. One way to deliver that force in the lab is by shaking the gel with a machine that vibrates at high speeds, but in theory there are lots of other low-tech options. “This experiment would work if you took a hammer and smashed it the whole time you’re trying to test it, but that’s laborious so we don’t do that,” Huang jokes.
While the gel is being shaken, it is connected to a device that measures electrical signals coming from the piezo-NPs. Huang compares the signals from gels soaked with different chemicals and identifies which of them allows the signals to travel farthest. She will then research rigid materials, looking for ones that have similar chemical properties to the most effective gel and therefore have potential as rigid self-strengthening materials.
Looking forward
Developing a rigid self-remodeling material is the aim of Huang’s graduate work, but she is already considering how the research could be built upon once she has met her goals.
“Further down the line, we want to be able to tune the spatial resolution, to adjust how much strengthening is happening and where,” Huang said.
This means being able to control how far the signals can travel, making self-strengthening materials customizable for all kinds of technologies from a pacemaker that could resist damage and keep a heart going longer, to an undersea pipeline that would better withstand pressure and require fewer repairs.
Huang has been working on this project for many months and progress has not always been made in a straight line. She has gotten unexpected results and has needed to change her approach to move forward. However, she finds figuring out how to navigate these challenges rewarding.
“I’ve enjoyed the process of learning how to be a better researcher,” said Huang. “I think that’s the biggest thing that I’ve gotten out of everything I’ve done so far.”
This dedication to the scientific process, as well as its results, make researchers like Sophia Huang more resilient in the face of inevitable surprises. With seemingly-endless applications for her work’s technology, it’s a good thing researchers like Huang are working to make them a reality.
By Lily Burton
Lily Burton is a PhD candidate at the University of Chicago in the Biochemistry and Molecular Biophysics program. She works in Michael Rust’s lab studying circadian rhythms in photosynthetic bacteria.
This article was written in collaboration with the myCHOICE Writing for Communications and Marketing Careers workshop, hosted by Marina Damiano of the Damiano group.